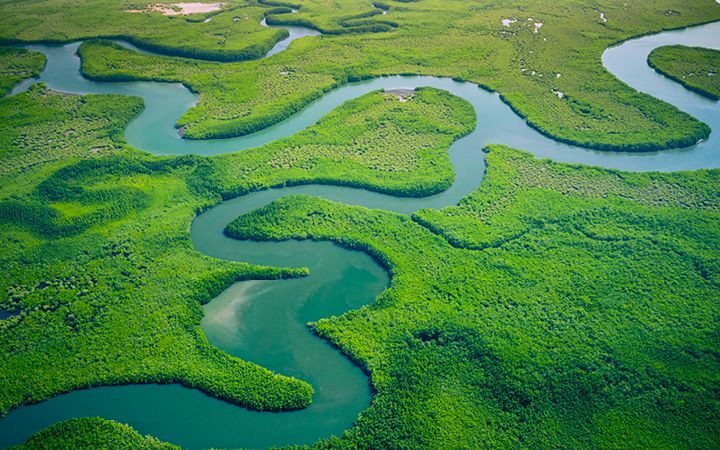
Pathways to Decarbonizing the U.S. Power Sector by 2035
Note: this article was written before the Inflation Reduction Act became law. You can read our analysis of the IRA's impact on clean energy costs here.
In April 2021, the United States submitted its updated nationally determined contribution (NDC). The NDC set an economy-wide target of reducing net greenhouse gas (GHG) emissions by 50%–52% below 2005 levels—in 2030 and included a goal of a 100% carbon-free power by 2035. Meeting these ambitious targets will require a transformational change to how power is generated in the U.S., and a rapid transition to low carbon and carbon-free resources.
In 2019, emissions from electricity generation comprised 25% of total U.S. GHG emissions (see Figure 1), mainly because of the use of fossil fuels. In the same year, about 37% of electricity in the U.S. was generated from carbon-free resources including solar, wind, geothermal, biomass, hydroelectric, and nuclear power.
Per the U.S. NDC, a carbon-free electricity grid “could be achieved through multiple cost-effective technology and investment pathways, each resulting in meaningful emissions reductions in this decade.” Ample opportunity to reduce emissions from the power sector exists. But making decisions about the technologies to deploy and investments to make while maintaining a reliable and resilient electric system is not a simple task.
Here we use an analytical framework to visualize and draw insights—leveraging our strategic decarbonization and clean energy planning platform, CO2Sight—about the challenges and opportunities associated with reaching a carbon-free electricity mix by 2035 under different future electric growth scenarios.
Key results at a glance
- 2 pathways analyzed that meet the 2035 goal
- 35 GW to 50 GW annual average battery storage capacity built over the next 14 years
- 11% to 30% increase in demand
- $1.2T to $1.6T in CAPEX investments for renewables and storage capacity
- 11% to 35% increase in average peak demand
- 765 GW to 1,000 GW of variable renewable resources needed by 2035
Challenges and opportunities for decarbonizing the power sector
Transitioning to a carbon-free power grid requires an understanding of how to balance clean generation resources and future electric demand. Balancing these two factors presents challenges, but also offers opportunities to create a clean, reliable energy future for the country.
Emerging clean baseload technologies
Clean baseload generation and long-duration (weeks and months) storage technologies will be needed to provide reliability and flexibility to a decarbonized grid. Battery storage technology is advancing and is increasingly cost effective but continues to have duration limitations. Solutions like carbon capture and sequestration (CCS), green hydrogen, and renewable natural gas (RNG) face multiple barriers and are currently cost-prohibitive to deploy at scale.
Widespread deployment of CCS in the power sector has not been realized in the U.S. due to economic and other barriers. While the technology exists, the relative cost of CCS—particularly in relation to renewable technologies—and the lack of policies and incentives to drive CCS adoption have slowed deployment. In particular, large-scale commercial CCS investments in the U.S. have not seen much success; since 2010, the DOE has invested in nine demonstration projects, only three of which have succeeded. The lone successful power sector plant—the Petra Nova CCS Facility at a coal-fired generating plant in Texas—was idled in 2020 due to unfavorable economic conditions. However, growing interest in CCS given its potential to produce blue hydrogen for use in the industrial sector could help this technology reach commercial viability by mid-century.
With declining costs and improving production efficiency, hydrogen is likely to play a central role in a decarbonized grid and a carbon-free energy system more broadly. The potential for green hydrogen is multifaceted, as it can be used directly for both electricity generation and as a long-duration storage medium to meet seasonal fluctuations in energy demand. Realizing the full potential of green hydrogen will require addressing multiple issues, including infrastructure requirements, regulatory and market barriers, and minimal policy support. Currently, most hydrogen is produced using natural gas, and the cost of implementing green hydrogen solutions must decrease and be incentivized for the technology to have the most impact on reducing greenhouse gas emissions. The U.S. lacks a comprehensive strategy for the development of green hydrogen, and the industry is currently focused on R&D and pilot projects. Hydrogen is likely to be utilized in the industrial sector in the near-term, and then move into other end uses such as power generation as the technology matures and production costs decline.
These types of barriers are only enhanced for other baseload technologies, such as synthetic gas (methanated green hydrogen), which also faces additional technical and implementation hurdles. However, some low carbon fuels offer near-term emission reductions, as well as form part of long-term solutions to achieve a decarbonized energy system. For example, RNG from sources such as landfills and animal manure are already fully commercialized and technologically mature. With multiple feedstocks from various waste streams, RNG has the potential to be a key source of low carbon baseload and flexible generation. Minimal policy support currently contributes to inhibiting the broadscale deployment of RNG, but with a more conducive regulatory framework that appropriately values the environmental benefits, RNG production could continue to grow both now and out to 2050.
Renewable technologies
Clean generation technologies such as solar and wind are well established, and in many cases have regulatory and policy frameworks in place to support them. But it’s not as simple as “turning on” resources like solar and wind as their availability is based on natural cycles and weather. Understanding the efficiency, diurnal availability, and reliability of renewable technologies are critical steps to making optimal decisions along the road to a decarbonized power sector by 2035.
Renewable technologies such as solar and wind are likely to be deployed in large quantities to achieve a carbon-free grid, but the speed and level of adoption of these technologies needs to be significantly ramped up in the coming years. The level of adoption will depend on policy mechanisms (e.g., Renewable Energy Credits or carbon prices), comprehensive and streamlined approaches to siting and permitting, and the evolution of flexible technologies and programs such as energy storage and demand response to help balance the intermittency of renewable generation. The duration and availability of energy storage will play a significant role in how much renewable generation can contribute to the 2035 goal.
Transmission requirements
The geography of the U.S. is diverse, and renewable resources are sometimes most available in remote locations that might not be close to dense urban areas or, in the case of offshore wind, directly offshore from load centers. Investments in the transmission system will be necessary to transport new renewable energy to population centers. Other transmission improvements—upgrades to decrease losses; grid-hardening strategies such as undergrounding transmission to increase resilience; and building new transmission lines to service projected demand increases due to increased end-use electrification (i.e., new load from electric transportation)—will also be necessary to transport electricity more efficiently and reliably.
These considerations translate to a need for states, RTO and ISOs, and federal regulators (e.g., FERC and DOE) to consider issues such as transmission upgrades, interconnection cost allocation, and the siting and permitting of new transmission. All will be crucial for building the regulatory structures necessary to set the stage for large-scale renewable development.
Flexible load strategies
A complementary strategy to integrate renewables and meet rising electricity demand is the development of flexible load solutions to accommodate and optimize the increasingly challenging supply-demand balance. Flexible load solutions include distributed solar, on-site battery storage, more targeted energy efficiency, and the implementation of temporal and geographical demand response strategies. These solutions can help can defer transmission and distribution (T&D) or centralized generation investments, help manage grid congestion, increase reliability for end users, and increase grid resiliency through a more flexible and bi-directional approach to power.
Planning for an increased amount of distributed energy resources (DER) has important implications across the energy system, and managing new load (e.g., electric vehicle charging and building electrification) will be important for managing system peaks. While many flexible load management practices are being adopted today, barriers remain to widespread growth and adoption, as well as for integration with utility operations. Regulatory and market changes will be needed to fully realize the benefit of load management strategies, such as market rules regarding DER aggregation into wholesale markets (which FERC Order 2222 is designed to address) and the creation of multi-day markets that value long-duration storage.
Climate resiliency
The recent winter storm in Texas, longer fire seasons in California, flooding across Appalachia and the Northeast, hurricanes in the Southeast and heat waves in the Northwest last summer all led to grid outages, and in some cases very extended outages that had ripple effects through the U.S. economy. These events point to (1) how dependent we are on electricity, and (2) the weaknesses of the current energy system to extreme weather events. For utilities to take meaningful steps toward implementing system resilience, they must meet several challenges, including understanding the vulnerabilities of their infrastructure and processes—ranging from worker safety to asset failure—and prioritization of available options to address resilience gaps both today and in the future.
Key stakeholders—including utilities, investors, state and local governments, and federal agencies—are beginning to take stock of the vulnerabilities of the electricity system and are attempting to address them. According to an ICF analysis, there is an additional capital investment gap of more than $500 billion needed to provide the level of resilience required for U.S. investor-owned energy utilities to effectively address risks from climate change by 2050 and prepare energy systems for a changing environment. This gap is driven by multiple sources of investment costs—outside of general operations and maintenance costs—across utilities aimed at protecting infrastructure and assets against climate-related hazards such as rising temperatures, extreme storms and flooding, sea level rise, extended drought conditions, and more frequent wildfires.
Planning for these investments alongside investments needed to decarbonize the grid will lead to a more optimal solution and smarter long-term choices. For example, increasing temperatures impacts generation, transmission, and distribution systems in several ways. Particularly, higher ambient air temperatures increase customer demand while reducing the efficiency of equipment (e.g., transformers and transmission lines). This combination of factors requires greater delivery capacity while making it harder for systems to remove heat. More frequent, severe, and longer heat waves also stress grid equipment and increase the risk of blackouts, requiring additional investments to strengthen system reliability. This situation will be essential to understand and plan for as new transmission infrastructure is to help facilitate a fully decarbonized U.S. grid by 2035.
Pathways to 2035
Reaching the U.S. NDC goal of a carbon-free grid by 2035 requires understanding the challenges and opportunities described above and developing solutions to address them. This is a complex problem to solve, but there are analytical approaches that can provide a more complete understanding of the tradeoffs and considerations of decarbonization strategies. The pathways presented below do not assume a specific policy mechanism to decarbonize the power sector, but to reach the U.S. NDC goal new federal regulations and policies will be needed both to incentivize the transition to clean energy and to ensure the necessary T&D infrastructure is in place.
Two pathways
We modeled two scenarios to illustrate a potential least-cost pathway to decarbonize the power sector’s generating mix, based on two future electric load growth scenarios. To do this, we leveraged the CO2Sight decarbonization planning platform—which harnesses decades of ICF’s experience designing energy and climate policies and programs, across sectors and technologies, into a unified pathways analysis environment.
The two pathways both include a carbon-free grid constraint by 2035; the focus is on examining different levels of energy demand over time to illustrate the impact that varying levels of efficiency and electrification of end-use sectors can have on the grid. The demand for electricity in our modeled pathways is based on the Electrification Futures Study (EFS) from NREL. The Reference EFS demand is used in our Reference pathway, while the High EFS demand is used in our Accelerated pathway. NREL’s High EFS demand forecast includes aggressive electrification and energy efficiency assumptions for the buildings, transportation, and industrial sectors.
The study’s demand reflects the impacts of energy efficiency, which has an increased impact in the accelerated electrification scenario but does not include load reductions from distributed energy resources such as rooftop PV. The Reference pathway we modeled is not a “business as usual” scenario, but rather represents meeting the U.S. climate targets for the power sector without the added complexity of considering potential impacts from decarbonization efforts in other sectors. These pathways are summarized in Table 1 below with results presented in Figure 2.
Figure 2. U.S. generation mix through 2040 for the Reference and Accelerated pathways
The generation mix shown in Figure 2 provides a representative view of one potential grid supply resource mix to meet varying levels of demand. In reality, different regions will rely on unique solutions based on their local grid conditions, market structures and policies, resource availability and electrification and efficiency impacts. This analysis does not consider specific breakthrough baseload clean energy technologies (e.g., advanced SMRs) that may emerge in the future; rather, a “low-carbon gas” option is modeled that reflects a mix of natural gas with CCS, green hydrogen, hydrogen storage, and renewable natural gas. While not reflected in the generation charts above, battery energy storage systems (BESS) are also modeled as a capacity resource. Transmission expansion is not modeled in this analysis, and therefore its costs are not included. However, the placement, timing, and amount of new transmission capacity could change when and where renewables are developed and will add to the overall cost of the energy transition. Transmission expansion costs to decarbonized the grid are expected to be significant—between $100 billion and $350 billion.
The generation mix for the two pathways above will take an incredible amount of planning and early investment to realize. The lead time from project planning to grid connection varies by the type and scale of project, but in general these graphs show that compliance with the U.S. NDC goal requires investment and project planning of renewables to ramp up immediately to create the resource mix shown. Investments in grid-connected resources must happen years before the plants come online as developers go through siting and permitting, interconnection, and other approval processes. In addition to generation investments, early transmission planning and investment is also critical; new transmission capacity will involve longer (sometimes decades-long) lead times and will need coordination between local, state, and federal government agencies for siting and permitting. A delay in transmission infrastructure development could limit renewable deployment and is a risk for realizing the U.S. NDC goals.
There is more to the story than what is presented in these pathways. If end-use sectors become increasingly electrified, as market trends are indicating, utilities, developers, regulators, and policymakers will have to work together across sectors to ensure that the grid can handle the influx of demand. This coordination goes beyond traditional grid planning. Electrification strategies in the buildings and transportation sectors must consider how to mitigate their impact on the grid. The traditional utility paradigms will need to shift to fit a more variable, flexible, bidirectional, and decentralized energy future while meeting reliability and resiliency standards that are also transforming to consider climate impacts and meet the challenges of a decarbonized energy system.
Key takeaways
From 2020 to 2035, U.S. national electricity demand increases by 11% in the Reference pathway and by 30% in the Accelerated pathway, while average peak demand increases by 11% in the Reference pathway and by 35% in the Accelerated pathway. Compared to the Reference pathway, 2035 average energy and peak demand are nearly 20% and 25% higher respectively in the Accelerated pathway.
A diverse mix of renewables and low-carbon baseload resources is required as part of a cost-effective, reliable, and resilient solution. This will mean a substantial transformation of the grid and significant investments to support it.
- In the Reference pathway, nearly 765 GW of variable renewable resources are required by 2035 to meet demand, four times today’s level of renewable capacity in the U.S., requiring $575 billion in investments and an average build rate of 40 GW per year.
- In comparison, renewables in the Accelerated pathway will have to grow by more than five times their capacity from today’s level to reach 1,000 GW by 2035, increasing the investment needed by $200 billion and raising build rates to an average of 50 GW per year.
- The total investment in CAPEX for renewables and storage capacity will surpass $1.2 trillion in the Reference pathway and increases to nearly $1.6 trillion in the Accelerated pathway. The majority of this investment will be for battery storage, followed closely by investments for new solar capacity (see Figure 3). Note: This is a low-end estimate that does not include interconnection costs or O&M (2021$).
- The nuclear fleet is maintained throughout the forecast, providing a critical clean baseload resource.
Managing peaks to ensure reliability and resiliency requires creative and flexible solutions.
- In the Reference pathway, average peak and energy demand both increase 11% from 2020 to 2035. Coupled with the quadrupling of installed renewable capacity, the demand increase results in significant battery storage capacity additions. By 2035, BESS comprises 30% of all electric generating capacity. This would require an average of 35 GW of BESS to be added each year over the next 14 years. In the Accelerated pathway, average energy demand increases by 30% from 2020 to 2035, and average peak demand increases by 35% in 2035, resulting in about 40% more battery storage compared to the Reference pathway to handle both the higher load and renewables deployment. In the Accelerated pathway, the BESS build rate would increase to 50 GW in each year.
- Load flexibility strategies can play a key role in helping to provide reliable power and reducing grid and consumer costs.
- Electrifying and decarbonizing from the perspective of implementing load management strategies—such as intelligent virtual power plants and grid-interactive buildings—can help create a more equitable, flexible, bidirectional grid while reducing peak impacts and grid congestion.
From 2020 to 2035, U.S. national average electricity demand increases by 11% in the Reference pathway and by 30% in the Accelerated pathway, while average peak demand increases by 11% in the Reference pathway and by 35% in the Accelerated pathway. Compared to the Reference pathway, 2035 average energy and peak demand are 20% and 25% higher, respectively, in the Accelerated pathway. The section below highlights several strategies for how the U.S. can reliably meet these demand projections with a decarbonized power supply.
A diverse mix of renewables and low-carbon baseload resources is required
Meeting increasing demand while transitioning to carbon-free power results in the significant deployment of new renewable resources. In both pathways, our modeling demonstrates that the most cost-effective solution is not to shift entirely to one resource. When considering other factors like reliability, resilience, and consumer costs, this need for a diverse portfolio becomes more pronounced.
The generating mix, on a percentage basis, does not significantly differ between the two pathways. However, the magnitude of the generating resources required increases significantly, which impacts the system-wide changes that will be needed (e.g., additional T&D infrastructure). In the Reference pathway, renewables will have to nearly quadruple their capacity from 2020 levels to reach 765 GW by 2035, which will require an annual average build rate of 40 GW. In the Accelerated pathway, renewables will have to increase by more than five times 2020 levels to reach nearly 1,000 GW by 2035, resulting in an average build rate of 50 GW per year.
In both pathways, renewables supply about 50% of the generating mix. In the Accelerated pathway, nearly 300 GW of low-carbon gas resources will be required by 2035, comprising 30% of the generating mix. These reliable resources complement the existing carbon-free baseload generation from nuclear and hydro facilities, which supply 20% of the generating mix. This significant capacity deployment will result in challenges that need to be addressed related to interconnection processes, competing needs for land, build-out requirements for transmission infrastructure, cost allocation, and regulatory and market structures.
Peaks management will be critical
The amount of electrification—and when it impacts the grid—will have significant implications for utility reliability planning and grid infrastructure development. Managing the new and varied peaks due to end-use sector electrification will be difficult, but load flexibility strategies can play a key role in helping to provide reliable power. In the Reference pathway, average peak and energy demand both increase 11% from 2020 to 2035. Coupled with the quadrupling of installed renewable capacity, the demand increase results in significant battery storage capacity additions. By 2035, BESS comprises 30% of all system capacity. This would require an average of 35 GW of BESS to be added each year over the next 14 years.
In the Accelerated pathway, average energy demand increases by 30% from 2020 to 2035 and average peak demand increases by 35% in 2035, resulting in about 40% more battery storage compared to the Reference pathway to handle both the higher load and renewables deployment. In the Accelerated pathway, the BESS build rate would increase to 50 GW in each year. The amount of battery storage required could be significantly higher if dispatchable clean energy technologies do not develop at a fast enough pace to supply near a third of energy demand by 2035.
Both short- and long-duration battery storage options will be required to help balance renewable capacity additions and provide reliable and resilient electricity to end-use sectors. To do this, batteries will also be needed at different points throughout the power system: grid-connected and behind-the-meter. For example, co-locating batteries with EV chargers can help mitigate not only their impact on grid demand, but also defer T&D upgrades that may have been required for a constrained area of the grid. Batteries co-located with EV chargers on-site at buildings can also be used to supply the building during times of peak demand—or even discharge to the grid. Reducing peak demand impacts is also critical for reducing emissions, since polluting “peaker” plants are generally dispatched during peak hours.
Electrifying and decarbonizing from the perspective of implementing load management strategies such as intelligent virtual power plants and grid-interactive buildings can help create a more equitable, flexible, bidirectional grid while reducing peak impacts and grid congestion. For example, load flexibility can help balance EV charging demands with building loads to minimize grid impacts in constrained networks like New York City. NYC’s strategies for electrifying transport and heating could nearly double peak demand by 2050 without major investments in energy efficiency and load flexibility. At the national level, more than 50% of households use natural gas for heating. If half of those households converted to electric heating, that would increase residential electric load by almost 30% compared to 2020, with impact on peak potentially greater and a transition from many summer peaking systems to winter peaking systems in parts of the country. Managing load impacts from electrification measures will require multiple strategies, including energy efficiency, flexible load management, and DERs to ensure grid reliability.
Moving into action
The scale and pace of what needs to happen in the 14-year time horizon to meet the U.S. NDC goal of a decarbonized grid by 2035 calls for immediate action: large investments and bold moves need to be made to increase clean generating resources, manage load through demand-side flexibility resources, build out the supporting infrastructure for T&D and new technologies (e.g., hydrogen), and enhance grid resiliency and reliability. This will not be achieved without new federal policies and regulations that will drive towards cleaning the grid and managing peaks and load.
The costs of the U.S. NDC goal for the power sector will be significant given the generation, transmission and distribution level investments required but there are also significant benefits to this energy transition. Decarbonizing the energy supply will lead to health and economic benefits, through improvements in local air quality, job creation and a decreased risk of extreme weather events due to climate change. Furthermore, by meeting the commitments outlined in the U.S. NDC, the U.S. would be doing its part to address global climate change.
Authors’ note: Utilities, policymakers, regulators, businesses, and government agencies need to create implementable roadmaps for the clean energy transition and determine the steps that need to be implemented to reach the U.S. NDC goal.
This article is meant to be illustrative, as many other factors would have to be considered when charting a more concrete path to 2035. These factors include reliability, resiliency, equity, health benefits, costs for consumers, local geography, and regulatory structures, which can all be addressed in a robust and comprehensive analytical platform like CO2Sight to help move from understanding to decisions and action.
CO2Sight harnesses decades of ICF’s experience designing energy and climate policies and programs—across sectors and technologies—into a unified pathways analysis environment ready to be customized to different needs. Screen at a high-level or take a granular look at sectors of interest. Compare alternative scenarios and building an optimized pathway to an end goal. Assess relative costs of integrated reduction solutions. Then educate external stakeholders and set priorities to make a business case and drive action.